What is special about microgravity?
One of the biggest holy grails of the space industry has been manufacturing in space. Earth’s gravity well ensures that transporting mass to space will still cost something, even if launch costs continue to fall. If a company can mass manufacture a product in orbit from raw material stock, then this product can be used, without the hassle of having to transport it out of Earth’s gravity well.
This is the main economic case for asteroid mining. If Mars will one day be settled, or if millions of humans are living in space stations sometime in the long term future, there has to be a system in place to process and create in space; there can be no dependency on a difficult system of Earth transportation.
Mass production of goods is difficult, even without the added difficulty of doing it autonomously, in space. Skeptics of space manufacturing often raise the question of what can even be built in orbit that cannot be built on Earth, for considerably cheaper? What is the rationale for spending money on science experiments in space, and what is there even left to discover? These are good questions, and this piece aims to explore some answers.
A few fundamental concepts
Humans weigh the way they do on Earth due to Earth’s special gravitational acceleration of 9.80665 m/s². Beyond Earth, this number is different, due to a myriad of factors. On the Moon, for instance, the gravitational acceleration is 1.625m/s², or about 16% of Earth’s gravity. This is the reason the Apollo astronauts had so much fun on the Moon; jumping got them much higher, and they felt weightless. Mars has about 38% of the gravity of Earth. No matter where you go in the solar system, galaxy, or universe, gravity is going to be different. Space itself is fascinating, because even though there is always going to be some gravitational pull anywhere in the universe, as an object gets further away from the mass it is orbiting, it experiences a minor effect of decreased gravity. This, along with the greater process of traveling at orbital velocity, is why the International Space Station feels the effect of microgravity; while there is no such thing as zero gravity, the forces on the station are such that objects experience weightlessness.
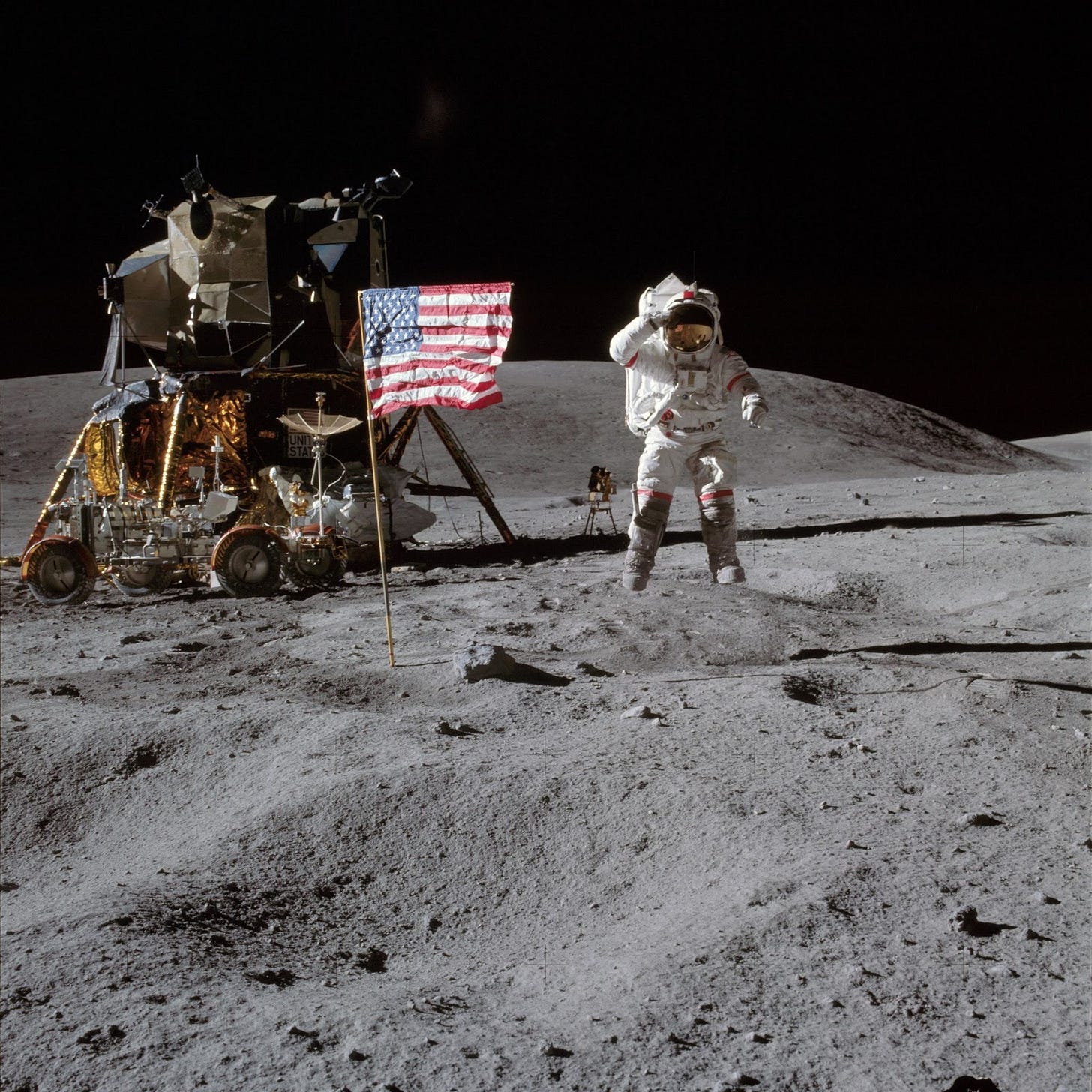
Gravity experiments on Earth are interesting. There are ways to increase the effect of gravitational acceleration. One of the best examples of this is my least favorite ride at the carnival, the Gravitron:
While there are ways to increase gravitational acceleration, there is currently no way on Earth to lower gravity. Some experiences, like parabolic flights, can simulate the experience for moments at a time, but to truly get the testbed of microgravity, ascending out of Earth’s immediate gravity well must be achieved. Applications for microgravity manufacturing (which will be discussed a bit later in this piece) need the full experience of microgravity, and as such, must be in orbit to fully capitalize on any breakthroughs.
Gravity is one of the four fundamental forces of physics. For all of time, humans have only had one number to experience: one g. Evolution has shaped us, and the world around us, with this number. However, most things work very differently when this force is altered. Tests on the International Space Station and other space stations have shown that applications of science, human physiology, and production techniques like welding, have a much more varied experience in microgravity versus the baseline on Earth. There is so much more still to learn from microgravity, but there are already benefits (and detriments to the human body) that have been discovered.
History
In October of 1969, the Soviet Union’s Soyuz 6 mission performed the first vacuum welding in space. Three methods of space welding were conducted: low pressure compressed arc, electron beam, and arc with a consumable electrode. Despite difficulties in the mission (and difficulties of performing in a weightless environment), the mission landed safely, and the experiments performed pushed the boundaries further. Four years later, NASA launched Skylab, the United States’ first research space station. While only shortly occupied, and not operational for long, a few missions were conducted to further test microgravity applications, including Earth materials experiments, more electron beam welding, and crystal growth (see Varda below). Post Skylab, the International Space Station (and China’s Tiangong Space Station) have carried out these types of experiments, among other activities.
With the International Space Station vastly surpassing its intended lifespan, there has been a push by the United States to have a successor, or successors, ready to continue orbital operations so that low Earth orbit is not ceded to China. Many nations around the world rely on the access to space that the International Space Station offers; if the ISS were to fall apart tomorrow, or if it were deorbited without a successor, there are scenarios where these nations might use Tiangong for their experiments, upsetting the current geopolitical status quo.
In their request for proposals on platforms to replace the ISS, NASA has stressed that it needs human-rated platforms to continue experiments. While the main selected companies working on building stations with these capabilities are pushing to meet deadlines of a possible 2031 deorbit date, none are without problems. Uphill battles in space industry finance and economics have brutalized the race to build human-rated stations. High capex costs and timelines that will not see profit margins for many years, if not decades, have crippled the field of contenders who want to build space stations, and have led investors away from investing in space station prototypes that focus primarily on human presence.
There may be a better way to do space stations. Varda is a space manufacturing company looking to produce pharmaceuticals and other goods in microgravity. Varda last year launched their first mission; their guiding mantra is that the missions fly on time, without delay on their end. While competing in a slightly different market area than human-rated space stations (at least at the moment), Varda has been able to launch faster and sooner than these other space station companies since they do not need to jump through the hoops of human spaceflight regulations.
By focusing on manufacturing and production, Varda can regularly launch missions that run autonomously. While human visitation is never off the table in these types of missions, by simplifying the process to a “minimum-viable-product” and launching immediately and often, Varda has been able to outcompete incumbent space industry players who are still in the weeds trying to launch large, complicated, expensive structures. By adopting a scrappy attitude, Varda has obtained a first-mover advantage for in-space manufacturing.
Materials
There are actually many benefits to creating goods in microgravity. First, space is an ultra vacuum; there is no risk of contamination from Earth particles, and potential laboratories in space will be cleaner than any clean room on Earth. The precise nature of this means that most objects produced will be free from defects, or will be crafted with less opportunity for defects caused by natural Earth forces. An absence of gravity makes it possible to build structures that would otherwise collapse in on themselves if produced in Earth gravity. Temperature control is also a benefit of space manufacturing; an object in shade can approach a temperature of absolute zero, while sunlight can be used to rapidly heat.
In the near future, semiconductor production could be moved up into orbit. There are drawbacks to this idea, with cosmic radiation being a potential threat. For instance, transistors operating in the space environment can experience total ionizing dose effects, which can alter the gates of the transistor, leading to issues in how they toggle their on/off positions. It remains to be seen if semiconductors that work better because they were produced in space will outweigh these drawbacks.
Optical fibers are another product that would see increased value from production in microgravity. Optical fibers are created via ZBLAN, an alloy of various metals including zirconium, barium, lanthanum, sodium, and aluminum fluorides. A problem with the current state of this process is that ZBLAN creates crystals which can scatter the signals and negatively affect the fiber itself. However, crystallization works differently in microgravity, and it would be possible to cool these fibers before crystallization can fully occur. Between this process, and the added benefits of microgravity manufacturing, optical fibers could be produced to be more efficient, and made longer, than the current baseline.
Keytruda is a drug that attaches to the protein DB-1 to assist in fighting off various forms of cancer. In 2017, Merck launched an experiment to the ISS to examine the effect of microgravity on the formation of Keytruda crystallization.
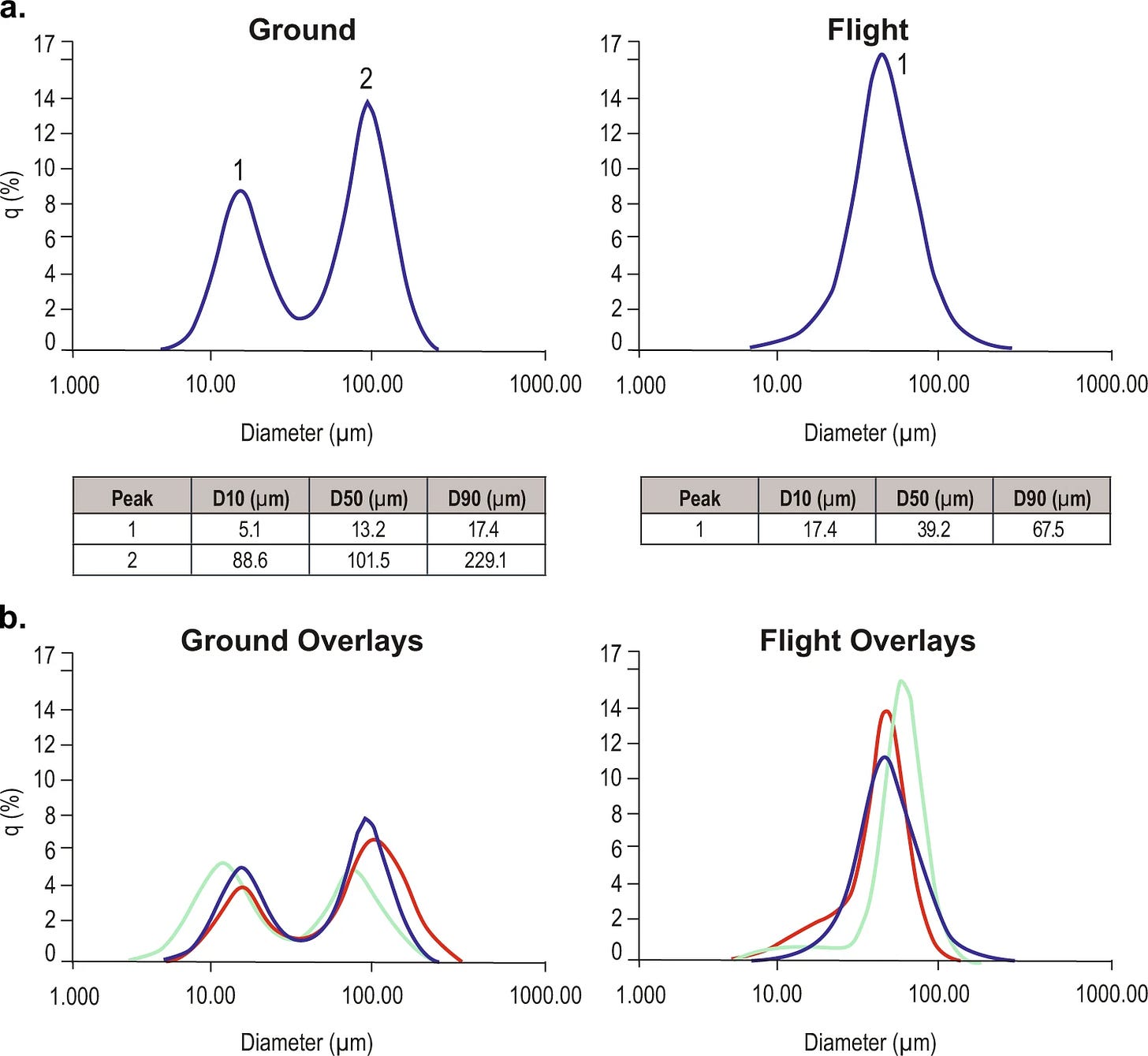
In June 2023, Varda launched their Winnebago-1 mission into orbit. Winnebago-1’s primary objective was to produce crystals of ritonavir, a drug used in the treatment of HIV. Upon landing, the product was retrieved and deemed a complete success.
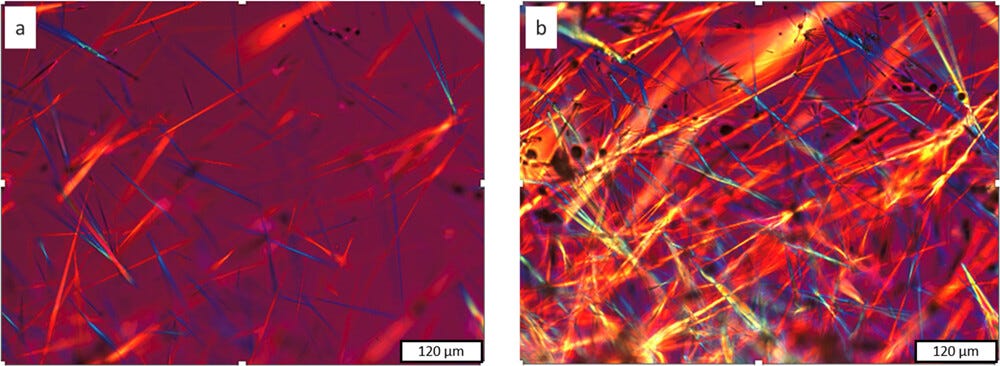
For future missions, Varda offers a teaser of what is to come:
Future work will examine polymorphic outcomes in microgravity by not only examining additional molecules, but also by expanding the range of thermal profiles examined, including probing behavior at the interface between known or anticipated polymorphic outcomes.
Science
Processes that are taken for granted on Earth should not be assumed to be the same in microgravity. One such example is the picture below, indicating the difference in a candle flame between regular gravity and in microgravity. This effect is due to an absence of convection flows in microgravity. On Earth, convection flows shape the flame into a longer form, and allow for soot to be carried to the top, which color it yellow. In microgravity, the lack of convection flows cause the flame to be spherical, and since soot is not carried upward, the flame is blue.
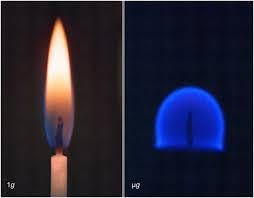
In 2015, NASA conducted one of the most interesting human experiments. Known as the twin study, this year-long endeavor saw Scott Kelly spend almost a year on the ISS, while his identical twin brother, Mark Kelly, stayed on Earth. There are many interesting results from this study, but perhaps the most interesting is the effect that this near-year spent in microgravity had on Scott Kelly’s telomeres.
Simply put, telomeres are structures at the ends of chromosomes that assist in the process of cellular division. These naturally shrink over time, but are essential to the inner workings of human physiology. Scott Kelly’s experience in space is so fascinating because his telomeres lengthened in spaceflight- they did not shorten, as would be expected. Even more interestingly, upon landing back on Earth, his telomeres rapidly shortened, and within a period of a few months, had fully shortened back to his pre-flight baseline. What this means is anyone’s guess, but it goes to prove that there is so much potential for an exploration of the effects of microgravity on nearly every aspect of human life.
Continued access and experimentation in microgravity is essential for acquiring knowledge for how to live in space. The effects of microgravity on human physiology, based on knowledge from years of ISS data, is not very promising, at least not at face value. Astronauts tend to experience bone density loss, muscle mass degradation, and other negative effects. Intuitively, this makes sense; human evolution has only ever had a gravity coefficient of one, and modern human physiology has adapted to need this very important aspect. Removing factors that are taken for granted can be very negative. There have been studies of astronauts in long-duration spaceflight experiencing hydrocephalus, which is probably explained by a lack of gravity to pull fluids through the body in the right way.
This shouldn’t be a deterrent to spaceflight, however. Humans are problem-solving machines, and with greater resources for studying these types of problems, we can master spaceflight. There is so much still to learn from how objects work in the microgravity environment. There are potential cures for many diseases hidden away behind future experiments, and these can be unlocked so long as the science experiments continue, without a gap in operational capabilities in low Earth orbit. If the United States and other countries embrace radical new ways of operating in orbit, both with human presence and also autonomously, they can push forward the banner of progress, maybe unlocking the secrets of human longevity and other universal mysteries.
At the very least, maybe we can figure out what exactly happened with Scott Kelly’s telomeres.
Thanks to Steve Newman, Sean Fleming, Rob Tracinski, and Rob L’Heureux for providing valuable draft feedback.